Underground Thermal Storage: The Industrial Energy Game-Changer You Need
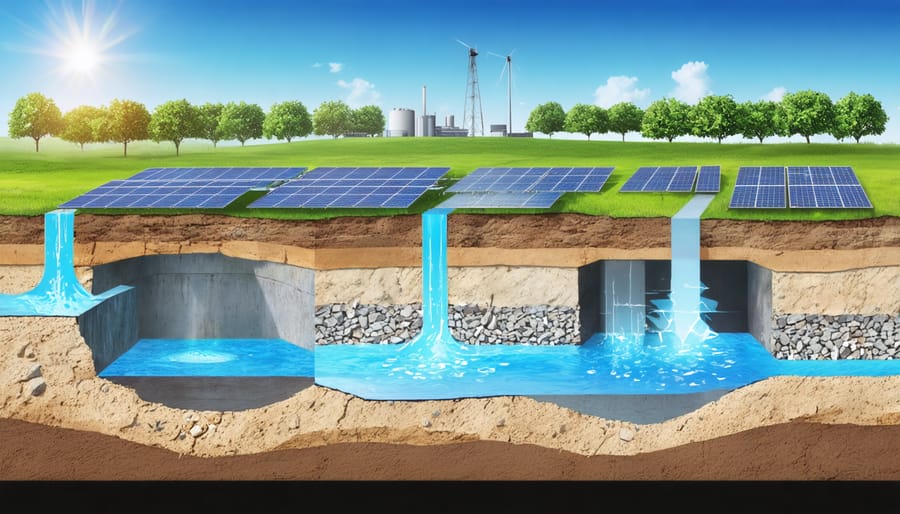
Revolutionizing renewable energy storage, thermal energy storage beneath the Earth’s surface represents one of today’s most promising solutions for sustainable energy management. By utilizing naturally insulated underground formations, this innovative technology can store vast amounts of heat energy during peak production periods and release it when demand rises, effectively bridging the gap between intermittent renewable generation and continuous energy needs.
European industries have already demonstrated the remarkable potential of underground thermal storage, achieving energy cost reductions of up to 40% while significantly decreasing carbon emissions. From abandoned mine shafts converted into thermal batteries to purpose-built aquifer systems beneath industrial complexes, these installations are transforming how we think about energy storage and distribution.
The technology’s versatility allows it to serve multiple sectors simultaneously – providing district heating for residential areas, process heat for industries, and seasonal storage for large-scale solar thermal systems. As Europe accelerates its transition toward renewable energy, underground thermal storage stands as a crucial enabler of this sustainable future, offering a proven, efficient solution for long-term energy management.
How Underground Thermal Energy Storage Works
Types of Underground Storage Systems
Underground thermal energy storage systems come in three main varieties, each suited to different geological conditions and operational requirements. Aquifer thermal energy storage (ATES) utilises naturally occurring groundwater reservoirs, storing energy by injecting heated or cooled water into these underground layers. This system is particularly effective in regions with suitable aquifer conditions, common across Northern Europe.
Borehole thermal energy storage (BTES) consists of an array of vertical boreholes, typically 50-200 metres deep, equipped with heat exchanger pipes. These systems are highly versatile and can be installed in most geological settings, making them popular for both residential and commercial applications throughout Europe.
Cavern thermal energy storage utilises large underground cavities, either natural or artificially created, to store hot water or steam. These systems offer massive storage capacity and are ideal for industrial-scale applications. Abandoned mines and salt caverns across Europe have been successfully repurposed for this technology, demonstrating innovative approaches to sustainable energy storage.
Each system type offers unique advantages in efficiency and implementation, allowing project planners to select the most suitable solution based on local geology, space requirements, and energy demands.
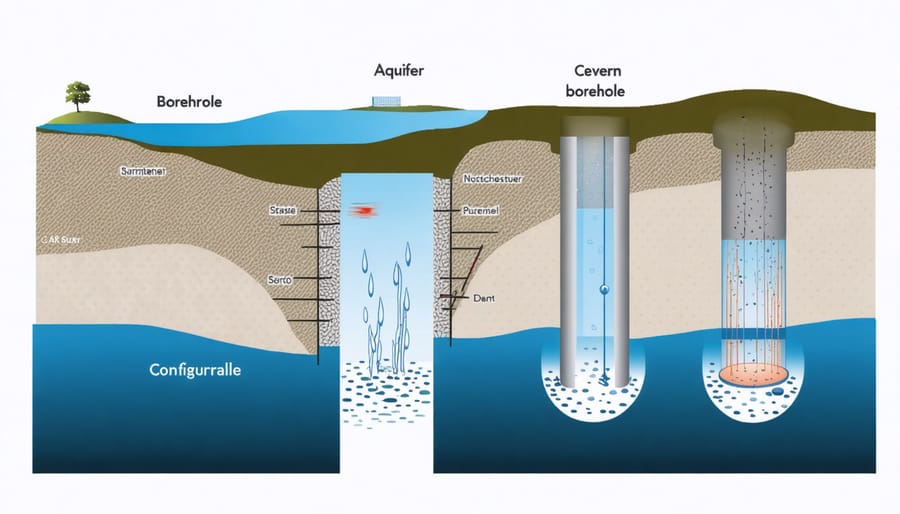
Key Components and Infrastructure
Underground thermal energy storage systems require several critical components for effective operation. At the core is the storage medium, typically consisting of natural geological formations such as aquifers, rock caverns, or soil layers. These formations must possess suitable thermal conductivity and storage capacity properties.
The heat exchanger network forms another vital component, comprising an array of pipes or wells that facilitate heat transfer between the storage medium and the working fluid. These systems often include both injection and extraction wells, strategically positioned to optimise thermal distribution and recovery.
The infrastructure also requires sophisticated monitoring and control systems to manage temperature levels, flow rates, and pressure conditions. Essential equipment includes circulation pumps, valves, sensors, and automation controls that ensure efficient operation and system protection.
Insulation plays a crucial role, particularly in the upper layers of the storage system, to minimise heat losses to the surrounding environment. Surface facilities house the necessary mechanical equipment, including heat pumps, circulation systems, and connection points to the energy distribution network.
Connection infrastructure links the storage system to heat sources (such as solar thermal collectors or industrial waste heat) and end-users through district heating networks or individual building systems.
Industrial Applications and Benefits
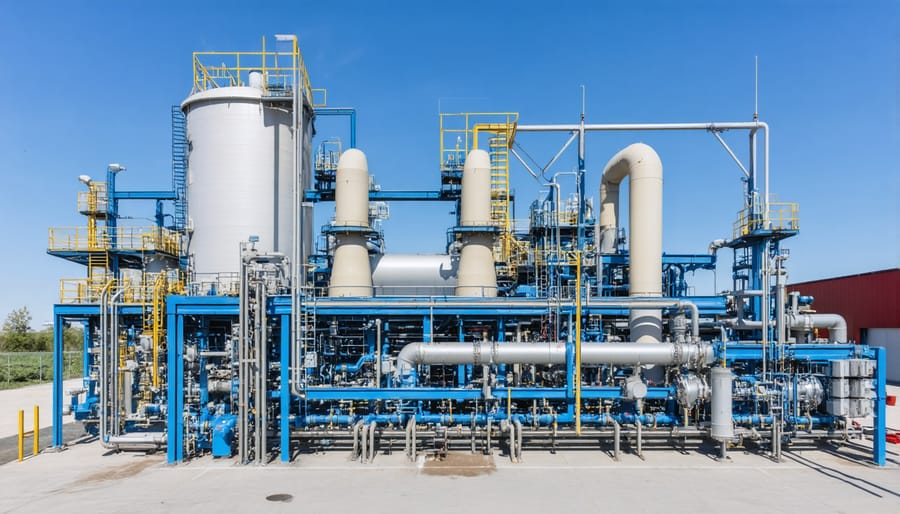
Process Heat Management
Process heat management in manufacturing and industrial applications represents one of the most significant opportunities for underground thermal energy storage (UTES) implementation. Industries with high thermal energy demands, such as food processing, chemical manufacturing, and textile production, can leverage UTES systems to optimize their industrial energy management strategies.
The system works by capturing excess heat generated during production processes and storing it underground for later use. This stored energy can then be retrieved when needed, reducing the overall energy consumption and operational costs. For instance, a food processing facility might store waste heat from sterilization processes during summer months and utilize it for space heating during winter.
European manufacturers have been particularly successful in implementing UTES solutions, with notable examples in Germany and Sweden. These installations demonstrate significant energy savings, with some facilities reporting up to 40% reduction in process heating costs. The technology proves especially valuable in continuous manufacturing operations where steady temperatures are crucial for product quality.
Integration with existing industrial infrastructure is typically straightforward, requiring minimal disruption to ongoing operations. The storage systems can be scaled according to specific industry needs, from small-scale batch processing to large continuous production lines, making them a versatile solution for various manufacturing sectors.
Energy Cost Reduction
Underground thermal energy storage systems offer substantial financial benefits through significant reductions in energy costs. Studies across European installations demonstrate ROI periods typically ranging from 4 to 8 years, depending on system scale and local energy prices. For industrial applications, these systems can reduce heating and cooling costs by 40-60%, contributing to energy independence for industries while stabilizing long-term operational expenses.
The cost-effectiveness becomes particularly evident during peak demand periods when conventional energy prices surge. A medium-sized industrial facility implementing UTES can expect annual savings between €50,000 and €200,000, with larger installations achieving even greater benefits. The system’s efficiency increases over time as the underground thermal reservoir stabilizes, leading to improved performance and reduced operational costs.
When combined with renewable energy sources like solar thermal systems, the financial advantages multiply. Users can store excess thermal energy during high-production periods and utilize it during peak-price hours, effectively creating a natural hedge against energy market volatility. Maintenance costs remain relatively low, typically requiring only 1-2% of the initial investment annually for system upkeep and monitoring.
Moreover, various European incentive programs and green energy initiatives can significantly offset initial installation costs, making the technology increasingly accessible to businesses and industries of all sizes.
Implementation Considerations
Site Assessment Requirements
Successful implementation of underground thermal energy storage requires thorough site assessment to ensure optimal performance and safety. The geological composition of the intended storage area plays a crucial role, with ideal conditions including stable rock formations or suitable soil types with good thermal conductivity.
Key geological prerequisites include adequate depth for storage, typically ranging from 50 to 300 meters, depending on the system type. The ground must have sufficient thermal conductivity and heat capacity, with crystalline rocks or water-bearing layers being particularly advantageous. Important factors include groundwater conditions, soil permeability, and the absence of geological faults or fractures that could compromise system integrity.
Technical assessments must evaluate the available space for both underground storage and surface installations. This includes drilling accessibility, connection possibilities to existing energy infrastructure, and distance to end-users. Environmental impact studies are mandatory in most European countries, focusing on groundwater protection and potential effects on local ecosystems.
A comprehensive site investigation typically involves:
– Geological surveys and core sampling
– Hydrogeological assessments
– Thermal response testing
– Ground stability analysis
– Environmental impact evaluation
– Local building regulations compliance check
These assessments should be conducted by qualified geologists and engineers to ensure project feasibility and optimize system design for maximum efficiency and sustainability.
Regulatory Framework in Europe
The regulatory framework for underground thermal energy storage (UTES) in Europe is governed by both EU-wide directives and national legislation. Projects typically require environmental impact assessments and permits from local authorities, with requirements varying by country and project scale.
In most European countries, developers must obtain drilling permits and underground usage rights before commencing UTES installations. These permits often involve geological surveys, groundwater protection assessments, and proof of technical feasibility. The Water Framework Directive plays a crucial role, especially for aquifer thermal energy storage systems, ensuring groundwater protection.
Property owners must comply with building codes and energy efficiency regulations, which increasingly encourage renewable energy integration. The Energy Performance of Buildings Directive (EPBD) influences UTES implementation, particularly in new construction projects and major renovations.
Several European countries offer streamlined permitting processes for residential and small commercial UTES systems. For example, Sweden and the Netherlands have established clear guidelines and simplified procedures for ground-source heat pump installations. Larger industrial projects face more rigorous requirements, including regular monitoring and reporting obligations.
Safety regulations typically mandate minimum distances from existing underground infrastructure, proper grouting procedures, and regular system maintenance. Additionally, many jurisdictions require certified installers and detailed documentation of system specifications and operational parameters.
Support mechanisms, including grants and tax incentives, are available in various European countries to promote UTES adoption, though eligibility criteria and benefits vary by region.
Integration with Renewable Energy Systems
Solar Energy Integration
The integration of solar thermal systems with underground thermal energy storage (UTES) creates a powerful synergy that maximises renewable energy utilisation throughout the year. Solar thermal collectors capture abundant solar energy during summer months, converting it into heat that can be stored underground for later use during colder periods. This combination effectively addresses one of solar energy’s biggest challenges: seasonal availability.
In a typical solar-UTES system, excess heat collected during sunny periods is transferred through heat exchangers into the underground storage medium, whether it’s aquifer layers, borehole fields, or pit storage systems. This stored energy can then be extracted during winter months or cloudy periods, providing a reliable heat source for space heating, domestic hot water, or industrial processes.
The efficiency of solar-UTES integration is particularly impressive in Northern European countries, where seasonal temperature variations are significant. For instance, projects in Denmark have demonstrated that solar thermal systems coupled with UTES can achieve solar fractions exceeding 50% of annual heating demands in district heating networks.
Modern control systems optimise the integration by monitoring various parameters such as solar radiation, storage temperature, and demand patterns. This intelligent management ensures optimal charging and discharging cycles, maximising system efficiency and extending the storage medium’s lifetime.
For building owners and industries considering solar thermal integration, careful sizing of both the solar collection area and storage volume is crucial. Success factors include proper geological assessment, system dimensioning, and implementation of efficient heat transfer mechanisms. The investment in combined solar-UTES systems typically shows favourable returns through reduced energy costs and enhanced sustainability credentials.
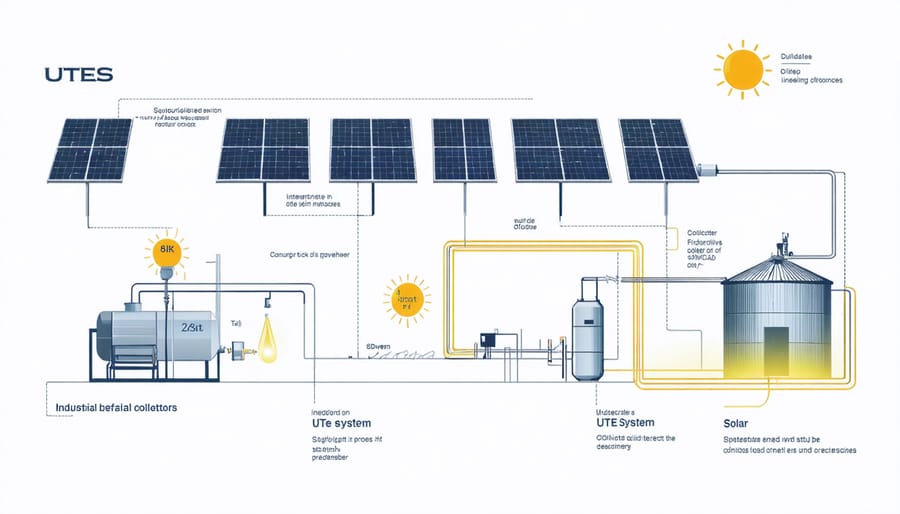
Underground thermal energy storage represents a pivotal technology in Europe’s transition to sustainable energy systems. As renewable energy integration becomes increasingly critical, UTES offers a reliable, efficient solution for managing seasonal energy demands. The technology has demonstrated its value across numerous successful implementations, from residential developments to industrial applications, proving particularly effective when combined with solar thermal and heat pump systems.
Looking ahead, UTES is poised for significant growth as energy storage demands increase and technology costs decrease. Innovations in drilling techniques, system monitoring, and thermal management continue to enhance efficiency and reliability. With supportive policy frameworks emerging across Europe and growing emphasis on carbon reduction, UTES systems are expected to play an essential role in future energy infrastructure. For businesses and homeowners alike, investing in UTES technology represents a forward-thinking approach to sustainable energy management while offering compelling long-term economic benefits.
Leave a Reply